Author: Jerome Kwon
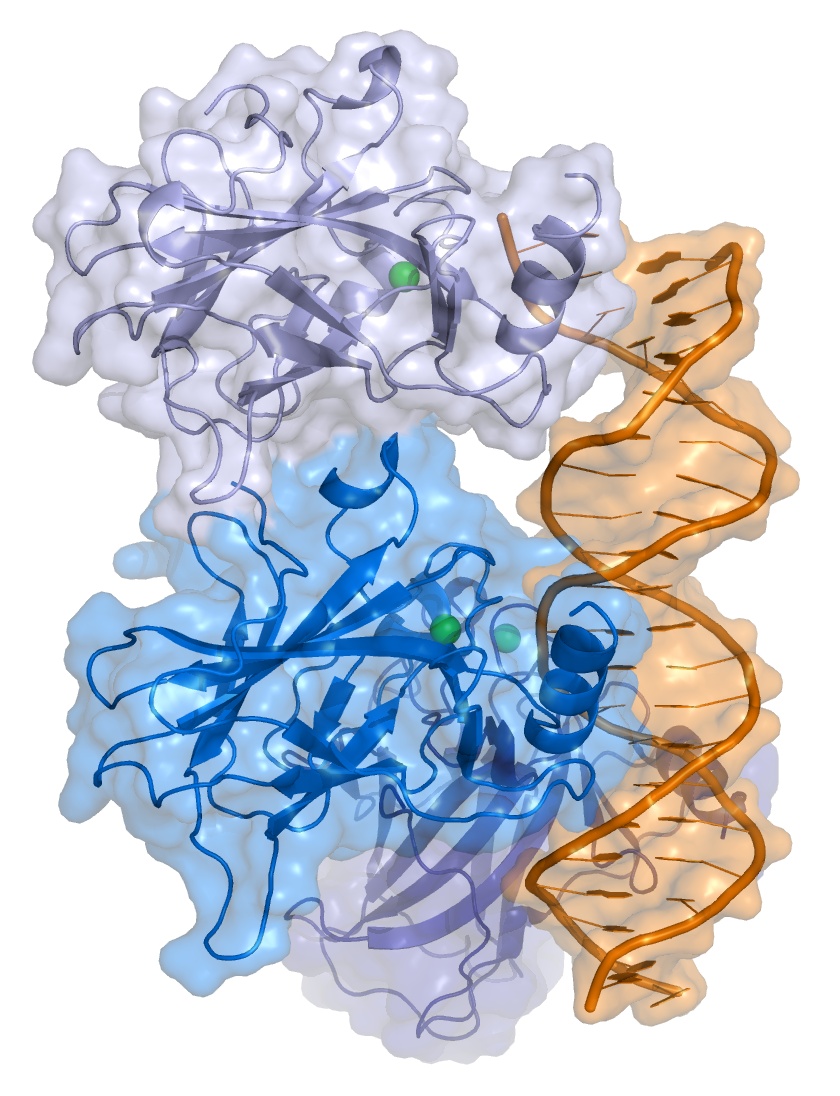
Abstract: P53, often referred to as the tumor protein, is a regulatory protein that plays a significant role in the human—and all other vertebrates’—body by preventing cancer formation. The mutation in the TP53 gene that encodes for p53 is shown to have a substantial effect on cancer formation, as it is mutated in about 50% of all cancer cases. In clear contrast to another key regulatory protein Rb, p53 is found in low concentrations in most cells under normal conditions. Hence, the p53 protein is only produced under a few circumstances, which are when, at the cellular level, pathological issues put the cell at extreme risk in function or survival. Such risks include DNA damage, telomere loss, hypoxia, osmotic stress, and oxidative stress. Under all of such situations, the cell is left with two options: a temporary halt of the cell cycle for recovery or a planned death—commonly known as apoptosis. The p53 pathway, in this situation, triggers an action from sensing such conditions, such as cell cycle arrest, senescence, or apoptosis. Cancer cells, which are indeed retarded, would not be allowed to proliferate if the p53 pathway is stable.
Cancer, at a cellular level, is defined by two properties. First, cancer cells reproduce in defiance of restraining factors of development. Second, they invade and reproduce in spaces of other cells. Commonly, cancer growth involves metastases, which spread within the body and may eventually cause a fatal failure of an organ, which makes cancer the leading cause of death in the modern world.
Normally, various factors control a cell’s growth and division. This includes both internal and external factors, such as kinases, hormones, proteins, etc. However, DNA mutation of the cell may disrupt such control and give rise to tumor formation. Therefore, regulatory pathways with the involvement of proteins p53, Rb and Ras regulate and respond to stress and mutation within the cell cycle, effectively removing dysfunctioning cells at most times through apoptosis. There are three key regulatory pathways that widely affect cell growth: the Rb pathway, the RTK/RAS/PI 3-kinase pathway, and the p53 pathway.
With regulatory proteins restraining tumor growth, it is clear that most cancer cases involve spontaneous mutations within the genes encoding for the regulatory proteins. Among them, p53 appears as the most prevalent, as a large number of cancer cases—about half, in fact—involve p53 mutation. A functioning p53 pathway responds to extracellular and intracellular signals by blocking the cell cycle. The factor of greatest influence is DNA damage, which may be caused from replication errors, chemical reactions, or radiation exposure. In order to stop tumor formation, it is necessary for the cell to repair such damage before proceeding through the cell cycle. Therefore, DNA damage initiates a signaling pathway, activating protein kinases ATM and ATR. ATM/ATR kinases phosphorylate multiple target proteins, including two other protein kinases, Chk1 and Chk2. These kinases target the gene regulatory protein p53, phosphorylating and activating it. The activated p53 initiates the transcription of CDKN1A, which encodes for p21, a Cdk inhibitor protein. Translated p21 binds to Cdk complexes and henceforth halts their activity, blocking the progression of the cell cycle. While other regulatory pathways such as the Rb pathway are consistently active in normal cells, p53 exists in low concentrations when the cell is undamaged. The protein Mdm2 causes this, which targets inactive p53 proteins to be ubiquitinated and degraded in proteasomes.
When extreme cellular stress or genetic mutation is applied, the p53 pathway acts like a siren that signals the cell to react in such an emergency situation. However, mutations in the TP53 gene may lead to the dysfunction of the p53 pathway. Unlike Rb and Ras, which predominantly face nonsense/deletion mutations that completely disable the protein, p53 is most mutated by a missense mutation—approximately 70 to 80% of p53 mutations change a single amino acid. Most of such missense mutations tend to occur at the DNA binding area of p53, inhibiting p53 from initiating the transcription of CDKN1A, thus leading to uncontrolled proliferation. Some mut-p53, unlike the majority of malignancies, go through a gain-of-function mutation. This may cause the mut-p53 to disrupt other cell functions such as AMPK and GLUT, possibly leading to uncontrollable cell proliferation.
In conclusion, p53 is a protein significant in cancer regulation. The p53 pathway is inactive in most normal cells, and is activated only when a cell is mutated or under stress. Because mutated proliferation may lead to malignancies, the p53 pathway responds by initiating apoptosis, senescence, cell cycle arrest, etc. However, when the TP53 gene that encodes for p53 is mutated, the p53 pathway dysfunctions, making the cell lose ability to take action against malignancies—which makes p53 prevalent in about 50% of all cancer cases. As current technologies are limited to the understanding of p53, future research may be done to specifically target p53 mutations for cancer therapy.
References
Alberts B., el al. Molecular Biology of the Cell, 7th edition.
Ashley M. Lakoduk, Cheng-Fan Lee, Ping-Hung Chen, Gain-of-“endocytic’ function in mutant p53 cancer cells, The International Journal of Biochemistry & Cell Biology, Volume 131, 2021, 105905, ISSN 1357-2725, https://doi.org/10.1016/j.biocel.2020.105905.
Lehninger A. L., et al. Principles of Biochemistry, 8th edition.
Mao, Y., & Jiang, P. (2023). The crisscross between p53 and metabolism in cancer. Acta biochimica et biophysica Sinica, 55(6), 914–922. https://doi.org/10.3724/abbs.2023109
Michael J. Duffy, Naoise C. Synnott, Shane O’Grady, John Crown, Targeting p53 for the treatment of cancer, Seminars in Cancer Biology, Volume 79, 2022, Pages 58-67, ISSN 1044-579X, https://doi.org/10.1016/j.semcancer.2020.07.005.
Rivlin, N., Brosh, R., Oren, M., & Rotter, V. (2011). Mutations in the p53 Tumor Suppressor Gene: Important Milestones at the Various Steps of Tumorigenesis. Genes & cancer, 2(4), 466–474. https://doi.org/10.1177/1947601911408889
Comments