Overview/Intro
No one imagined having computers and i-phones in the 80’s or in the 90’s. But now in the 21st century, we do. As such, the on-going developments of technology brought many benefits to our society. Despite its negative side effects, it has brought many opportunities to a diverse range of industries. Especially for the medical industry, the development of gene editing brought new opportunities for people to evade the consequence of hereditary diseases. In this article, one of the most powerful tools of gene editing (CRISPR-Cas 9) will be explained in detail, especially in relation to curing the fatal sickle cell disease.
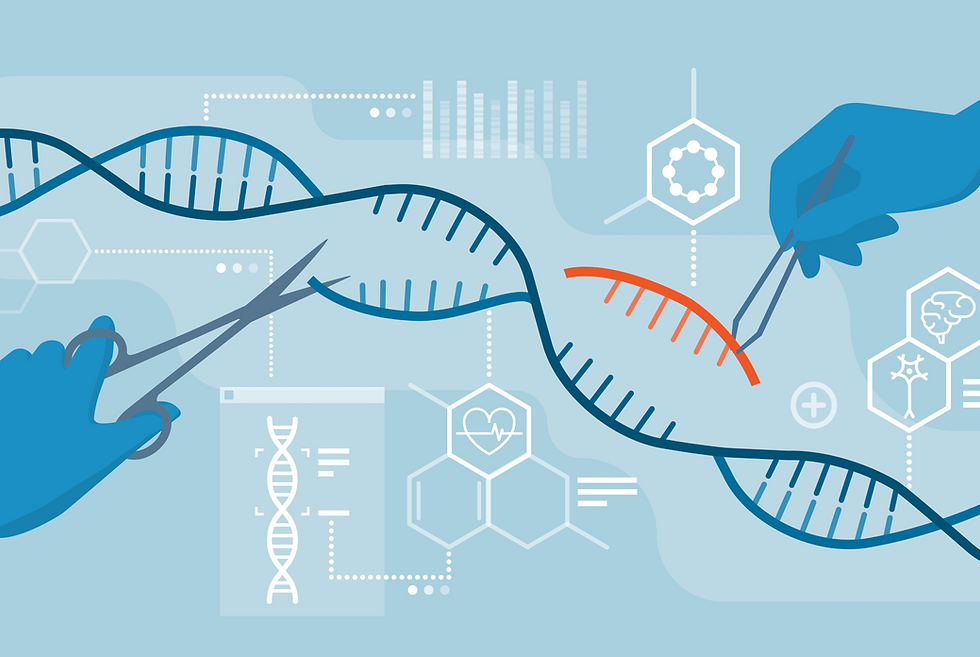
Author: Yebin Yun
Editor: Minjae Shin
What is a Gene?
To simply put, a gene is the basic physical and functional unit of heredity that is made up of many (a few hundred to two million) deoxyribose nucleic acids (DNA). Exons are the specific proportion of DNA that is used for encoding proteins, including some that are essential to our metabolic system. However, not all parts of the gene codes for the proteins -- these non-coding regions are called introns ("What Is a Gene?").
What is Gene Editing?
With many new technologies rising across the world, its advancements brought numerous changes to the discoveries of the ‘unknowns’ in the scientific community. One technology that is representative of this new impact is gene editing. Gene editing is “a group of technologies that give scientists the ability to change an organism’s DNA by allowing “genetic material to be added, removed, or altered at particular locations” in the gene. There are many different approaches to gene editing, including restriction enzymes, zinc finger nuclease, transcription activator, base editing, prime editing, and many more, which essentially aim to ‘edit’ the gene for therapeutic or research purposes. However, out of all those gene editing methods, CRISPR-Cas9 has shown to have the most potential to enhance gene editing discoveries.
The Invention of CRISPR-Cas9
The molecular tool of CRISPR-Cas9 (clustered regularly interspaced short palindromic repeats and Cas9 - the CRISPR associated protein) was discovered by an American scientist named Jennifer Douna, a French scientist named Emmanulle Charpentier, along with their colleagues in 2012. The mechanism of the CRISPR-Cas9 was inspired by a bacteria’s immune defense system. When a virus infects the bacteria, it captures small pieces of the viruses’ DNA and inserts them into their own DNA in a particular pattern to create segments known as CRISPR arrays ("What Are Genome"). These arrays then allow the bacteria to ‘remember’ the viruses that are closely related. In this sense, when similar viruses attack again, the bacteria would be able to defend itself by using CRISPR arrays to produce RNA segments that recognize and attach to specific regions of the viruses’ DNA. Subsequently, the bacteria uses Cas9 or a similar enzyme to cut the DNA apart, which disables the virus.
Why CRISPR-Cas9?
Now you may wonder, but why CRISPR-Cas9? To simply put, it is known to be the fastest, cheapest, and most precise technology for gene editing in the scientific community. To elaborate upon this claim, there are several necessary comparisons to make with RNAi (also a famous gene editing method that was discovered in the same period as CRISPR-Cas9). “RNAi silences genes by generating knockdowns at the mRNA level, while CRISPR generates knockouts at the DNA level” (Prabhune). Thus, in comparison to RNAi, CRISPR-Cas9 is an effective tool for ceasing the gene expression completely with fewer off-target effects than RNAi. Even though the reversible nature of RNAi has its own applications, the higher precision CRISPR-Cas9 provides to scientists puts them at a competitive edge. As a result, in the upcoming future, many scientists are assuming that the advancements of CRISPR-Cas9 is likely to replace RNAi for most of the research applications.
Application: Sickle Cell Anemia and CRISPR-Cas9
Sickle Cell Anemia (SCA) is one of the most infamous diseases that affects millions around the world. According to HCP Live, “300,000 babies are born with sickle cell disease each year in sub-Saharan Africa'' and many won’t live up to 5 years of age (Grossi). Being designated by the World Health Organization (WHO) and United Nations (UN) as a global public health problem, many scientists continue to research its molecular cause for its cure.
To further investigate its ‘molecular cause’, SCA occurs by a mutation of a single base in the DNA sequence of ß-globin gene (HBB). As a result, contrary to healthy individuals who have glutamic acid (GAG) in position six, SCA patients have valine (GTG) instead. This mutation results in the formation of hemoglobin S, the disease-associated form of the protein. Thus, the deoxygenated hemoglobin S distorts the conformation of the red blood cells which further causes it to lose its function as an effective oxygen-transporting molecule. In consequence, there is a shortage of red blood cells that cause recurrent pains and infections in the body ("Sickle Cell").
In an attempt to cure SCA, there are solutions such as the transfusion of blood with normal adult hemoglobin. However, CRISPR presents an alternative approach that is much more effective than transfusion - especially as it is a sensitive process for the blood to match the patient receiving it.
The CRISPR sickle cell gene therapy targets to repair the mutation in the adult hemoglobin gene that is responsible for sickle cell disease. To specify upon this approach, a research instructor named Daniel Dever in Matthew Porteus’ lab at Stanford University uses CRISPR-Cas9 to introduce a DNA break to the ß-globin gene. What this means is that a donor template containing the normal sequence of the gene will be introduced in order to correct the mutations when the cell repairs the DNA break with the template via homology-directed repair (HDR). Thus, the resulting edited cells that are re-implanted in the patient’s bloodstream will produce normal hemoglobin. The methods and the results of Dr. Dever’s preclinical development proves to be successful as it is incredibly efficient and reproducible by allowing for mutation and analysis of precise genomic locations to be completed in weeks. Dr. Dever plans to move forward with the CRISPR sickle cell gene therapy into clinical trials so that it can be implemented in actual circumstances.
Works Cited
Grossi, Giuliana. "New Estimate for the Risk of Child Mortality Due to Sickle Cell Anemia in Africa." HCP Live, 8 Mar. 2022, www.hcplive.com/view/new-estimate-risk-child-mortality-sickle-cell-anemia-in-africa.
Mah, Amanda. "Genome Editing Techniques: The Tools That Enable Scientists to Alter the Genetic Code." Synthego, www.synthego.com/blog/genome-editing-techniques#7-gene-editing-techniques-tools-to-change-the-genome.
Prabhune, Meenakshi. "RNAi Vs. CRISPR: Guide to Selecting the Best Gene Silencing Method." Synthego, www.synthego.com/blog/rnai-vs-crispr-guide.
"Sickle Cell Gene Therapy Using CRISPR." Synthego, www.synthego.com/crispr-sickle-cell-disease#approaches-to-crispr-sickle-cell-disease-gene-therapy.
"What Are Genome Editing and CRISPR-Cas9?" MedlinePlus, medlineplus.gov/genetics/understanding/genomicresearch/genomeediting/.
"What Is a Gene?" MedlinePlus, medlineplus.gov/genetics/understanding/basics/gene/.
Comments